Research
1) 3D Microfluidic Networks Formed by Sacrificial Melt-spun Microfibers
Video Player
00:00
00:00
While fabrication of 2D microfluidic structures has become a standard lab technique, the fabrication of 3D fluidic microstructures is still nontrivial. Typical processes for fabricating 3D structures include multilayer assembly of 2D structures, stereolithography, or 3D printing. While these techniques allow for exquisite control over the location of features, they are expensive, difficult, time-consuming, and often limited in the minimum feature size they are able to create. However, for many applications, precise placement of features is unnecessary, and it is simply the average feature size and geometry (aspect ratio, density, interconnectivity) that dictate the material properties. Thus, as a high-speed alternative to the more traditional 3D fabrication approaches, sacrificial melt-spun microfiber networks (“cotton candy”) are used to produce a 3D microfluidic network. Larger sticks of material can be “welded” to the microfiber network, producing macroscale inlet and outlet channels confluent with the microchannel system once sacrificed. This technique is applicable to a wide range of materials, is easily scalable, inexpensive, and rapid. We are employing these structures to engineer microvasculature in thick tissue constructs, and to enable novel large molecule drug delivery strategies.
2) Thermoresponsive transient electronics
Most electronics platforms are stable and invariant; they are designed to exist and function forever. Transient electronic systems represent a new class of electronics architecture that do not possess the long-term stability of typical printed circuit boards and silicon integrated circuits. Instead, these systems are purposefully engineered for finite functional lifetimes, after which the entire system disintegrates and stops functioning. We are developing a new class of transient electronics that exist and function in warm water, but disintegrate and irreversibly lose function in cold water. Thus, the system requires a constant source of heat to stay viable; when the heat source is turned off, the local environment cools and the electronics dissolve and disappear.
Video Player
00:00
00:00
3) Polarized human brain organoids
Human brain organoids derived from induced pluripotent stem cells (iPSCs) have tremendous potential to enable complex models of human brain development and neurodegenerative diseases. We are developing microfluidic approaches to expose such organoids to gradients of morphogens in order to achieve enhanced regional specification and dorsoventral or anteroposterior polarization, enabling higher complexity in these cutting edge platforms. 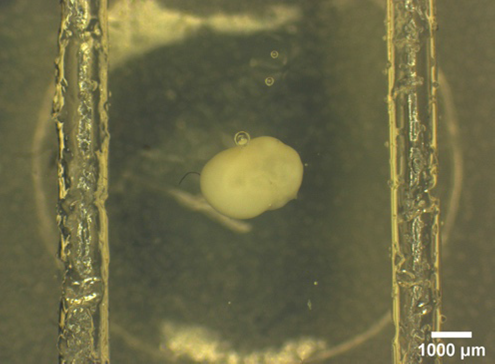
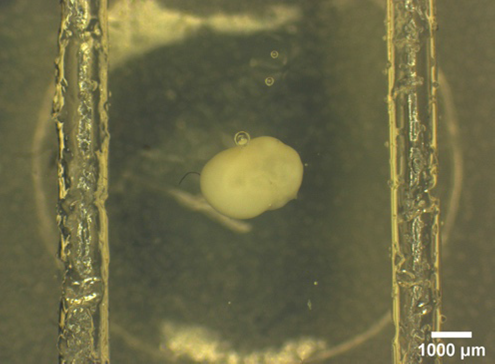