Home » News » Intraopertive Spectrally Encoded Coherence Tomography and Reflectometry (iSECTR) for imaging ophthalmic microstructures
Intraopertive Spectrally Encoded Coherence Tomography and Reflectometry (iSECTR) for imaging ophthalmic microstructures
Posted by anderc8 on Wednesday, September 27, 2017 in News, TIPs 2015.
Written by graduate research assistants Mohamed El-Haddad, Joseph Malone and Tom Manuel
The Diagnostic Imaging and Image-Guided Interventions (DIIGI) lab is located in the Vanderbilt School of Engineering and is part of the Vanderbilt Institute for Surgery and Engineering (VISE) and the Vanderbilt Biophotonics Center (VBC). Led by Dr. Yuankai (Kenny) Tao, our lab focuses on using light to visualize ophthalmic structures for research, diagnostic, and intraoperative purposes. We have four graduate PhD students (Mohamed El-Haddad, Ivan Bozic, Joe Malone, and Tom Manuel) that perform research ranging from the design of next generation optical coherence tomography (OCT) imaging systems to the development of computational image processing software for the visualization and identification of retinal vasculature in zebrafish.
We are continuously designing and building new systems to address limitations from older designs, or to evaluate novel ideas. Almost every design goes through a cycle of simulation using a combination of computer-aided design (CAD) software, rapid prototyping, and testing. The lab is well-equipped to streamline this process as much as possible. Besides the powerful workstations and the usual components you would expect to find in a standard optics lab, there is also a high-resolution 3D printer, and a machining room that houses a high-end milling machine. This allows us to rapidly assess system performance, and user experience and ergonomics. The latter is crucial because the nature of our research is directly translatable to clinical practice, and in addition to our lab, we have a unique opportunity to collaborate with physicians at the Vanderbilt University Medical Center. In one of our projects, we are collaborating with Dr. Karen Joos and Dr. Shriji Patel to develop an enhanced multimodal imaging system for surgical guidance using intraoperative Spectrally Encoded Coherence Tomography and Reflectometry (iSECTR), which will be the focus of this blog post.
OCT is the optical analogue of ultrasound; it utilizes low-coherence interferometry to measure echo time-delays of light waves for the reconstruction of high-resolution (1-10 um) images of subsurface tissue structures. The non-invasive, non-contact nature of OCT makes it ideal for ophthalmic imaging and it has become one of the most important diagnostic tests in standard clinical practice.

Left: Schematic of human eye and retinal cell structures. The cornea and the lens form the eye’s focusing elements. Light is focus on the retina, which is a multi-layered structure at the back of eye which contains the photoreceptors, and transmit visual signals to the brain via the optic nerve. Right: OCT cross-section labeled in tandem to a histological sample, confirming the ability of OCT of to resolve all the retinal layers.
A subset of OCT research focuses on harnessing this visualization power in the ophthalmic surgical suite. Progressive ocular diseases usually require surgical management at advanced stages, which involves precision manipulation of delicate semi-transparent structures in the eye.
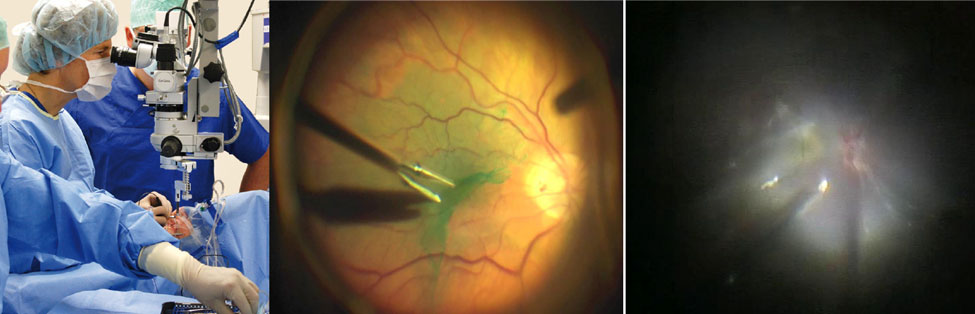
Left: Photograph of live ophthalmic surgery using a standard white light surgical microscope (Oculus GmbH, 2015). Middle: A pair of forceps peeling an epiretinal membrane stained with indocyanine-green. The surgical view is inherently two-dimensional and limits visualization of subsurface features of interest. Right: Visualization can be further obscured by the presence of scar tissue as in this complex case of proliferative diabetic retinopathy.
Limited visualization of these tissue layers remains a critical barrier to improving clinical outcomes and developing novel surgical techniques. While recent clinical iOCT studies have shown successful imaging of surgical field-of-views (FOVs), they add time to surgery and do not provide the dynamic information necessary for predicting postoperative function nor do they allow for comprehensive analysis of the clinical value of iOCT-guided surgical decision-making. Limitations of state-of-the-art iOCT systems include: (1) Static FOVs that require manual instrument-tracking (2) High frame-rates are achieved by sampling a small FOV which limits the area of visualization; and (3) small iOCT FOV also limits the number of anatomical fiducials (vasculature) and ability to co-register data with microscopy. Thus, the surgeon is forced to estimate the location of features-of-interest, identified using iOCT, on the microscopy field.
Our lab aims to address these limitations using iSECTR. Briefly, the system combines OCT cross-sectional imaging with en face reflectance images using spectrally encoded reflectometry (SER). SER utilizes line-illumination, which results in 1-2 orders of magnitude faster acquisition rates compared to point-illumination systems, and employs partially-coherent (quasi-confocal) detection to enhance collection efficiency and signal-to-noise ratio with increased depth-of-focus. The system illumination and detection engine and optics are designed such that OCT and SER acquisition are synchronized in time and space. This gives full three-dimensional information about sample motion, simplifies localization and registration with the surgical field, and enables more efficient sampling of the field.
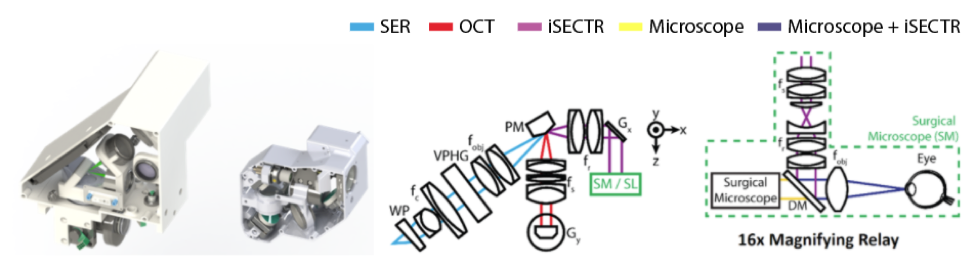
(From left to right) CAD renderings of first and second generation iSECTR scan-head modules with internal optics. Schematic of the iSECTR imaging optics using a prism mirror (PM) to combine the OCT and SER paths while reducing overall form factor. Schematic of the optical relay designed to integrate the scan-head optics into the existing optical pathway of the surgical microscope. WP: wedge prism, fc: collimating lens, VPHG: diffraction grating, PM: prism mirror, Gx,y: galvonometer, fs: scan lens, DM: dichroic mirror, fobj: objective lens.
While the system has been mainly developed for surgical guidance, it has applications in clinical diagnostics and potentially outside ophthalmology as well, since it enables acquisition and reconstruction of densely-sampled and anatomically accurate volumes. Thus, we designed and built a handheld probe which can also be mounted to commercial slit-lamp bases. Shown in the above figure are CAD renderings of our first and second generation handheld systems. The second generation probe reduced the overall size of SECTR by a factor of 3, providing enhanced ergonomics for clinical use.
A scan-head was also designed and prototyped to integrate iSECTR optics with the surgical microscope, as shown below.
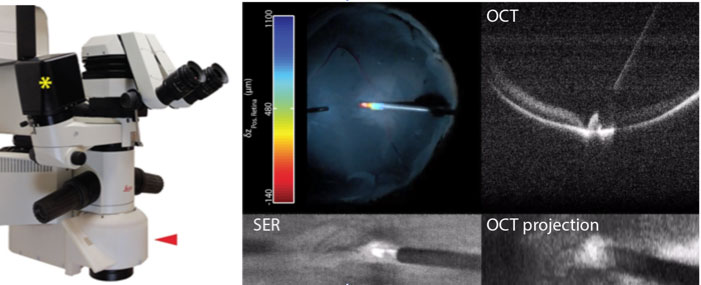
iOCT surgical scan-head and integrated microscope optics (red arrow) attached to a Leica surgical scope. Axial position color overlay of membrane scraper performing retinal sweeps and compressions was registered to the surgical view using SER images and calculated from OCT cross-sections.
Microscope-integrated iSECTR enables visualization of the surgical view of the microscope along with simultaneously acquired iSECTR frames. The above figure shows an example of this multimodality visualization in a simulated surgery performed on a cadaveric porcine eye. By segmenting the instrument and the retina from the OCT frames, we calculate the axial distance and encode it as a color channel, which is registered to the microscope view using SER frames, and overlaid on the surgical display in an augmented-reality fashion.
The overarching goal of this project is to provide enhanced, depth-resolved visualization of instrument-tissue interactions, and to enable quantitative analysis of the effect of surgically induced tissue morphology on visual outcomes. This may facilitate the development of novel, more complex surgical maneuvers that were not previously possible. Additionally, by leveraging the vast amount of information provided by this platform, combined with automated image segmentation and processing algorithms, and the recent developments in machine learning and parallel processing, we may enable computers to “understand” the surgical field, and facilitate transition to ophthalmic robotic surgery.
Learn more about VISE by visiting www.vanderbilt.edu/vise and follow us on our social media channels:
Facebook: https://www.facebook.com/visevanderbilt
Instagram: https://www.instagram.com/visevanderbilt/
Twitter: https://twitter.com/ViseVanderbilt
YouTube: http://vanderbi.lt/viseyoutube